Submitted by Dennis Bushnell on
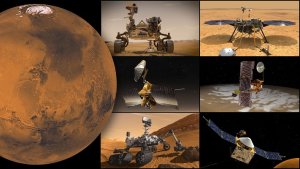
NASA's Mars missions, clockwise from top left: Perseverance rover and Ingenuity Mars Helicopter, InSight lander, Odyssey orbiter, MAVEN orbiter, Curiosity rover, and Mars Reconnaissance Orbiter. Credit: NASA/JPL-Caltech
Space business is currently approaching $500 billion a year and is composed of space science, national security space, humans in space, satellite communications, and imaging from space. These efforts are almost wholly “space for Earth” programs with strong business cases, becoming ever more capable and affordable due to the large ongoing decreases in costs of space access and to miniaturization.
Due to the huge additional costs of humans in space, almost all of space has been automated, and now with the developments in artificial intelligence (AI) and nano, it is moving to autonomous robotics. These recent, in-progress technologies are reducing costs to where there is increasing study, interest in space for space, space business beyond geostationary orbit (GEO), sometimes termed “deep space.” Also, companies have become proficient in space to not only develop and execute programs, including humans in space programs, but also to plan and operate them.
In regard to space science, national security space, and humans in space activities, where historically the government has been the customer and government entities the planners and operators, government labs are now becoming less competitive with industry and commercial entities. Some of the current deep space efforts are funded privately from other portions of the company business, sometimes for avowedly altruistic reasons and sometimes as investments in the future.
The stated goals of the evolving deep space for Earth and space efforts include finding life; working on unsolved problems in cosmological physics; developing defensive/offensive operations and sensing; advancing in-space resource utilization (ISRU), especially in regard to water for life support, radiation protection, fuel, and materials; achieving eventual colonization; and making profits.
The major current overall closed business case approach for deep space commercialization is planning, development, and execution of government programs. There are ongoing efforts to ideate or develop other potential deep space closed business cases as discussed herein. These are impacted by the much longer than usual time for maturation of these putative markets. There are serious issues that need to be addressed to improve the deep space market outlooks. These include human health and safety in space (especially galactic cosmic radiation, or GCR, and micro- or reduced gravity), costs, safety and reliability, power and energy, space debris, detailed information in regard to space resources, and trusted autonomy.
What Is Out There?
What’s out there is vast to unimaginable space(s), vacuum, low temperatures, solar energy, solar and GCR radiation, micro- or partial gravity, minerals, volatiles, oxides, etc., on planets, moons, asteroids, meteorites, dust, magnetic fields, photons, gravity fields, “position” (the source of the economic and societal value of the current commercial space for Earth, industry at GEO and below), interstellar space, the rest of the galaxy, and universe.
The number of asteroids, many of which are in the asteroid belt past Mars, is estimated at 150 million. Their diameter ranges from 33 feet to 530 miles. Their total mass is less than that of the Earth’s Moon. There are approximately 15,000 near-Earth asteroids, with 900 of them over a kilometer in size. Asteroids are classified as C type (chondrites), S type (silicates), and M type (metals) and can contain water and many other elements.
In terms of space object composition, aside from Earth, we know the most about the Moon and Mars. Resources available on Mars include nickel, titanium, iron, sulfur, magnesium, calcium, phosphorus, chlorine, bromine, aluminum, silicon, oxygen, hydrogen, carbon, nitrogen, sodium, manganese, potassium, chromium, deuterium, aluminum, lithium, cobalt, copper, zinc, niobium, and tungsten.
Currently, interstellar space beyond the solar system, with its now apparent multitude of planets, many of which are Earthlike, is limited to virtual travel because the distances are simply too far for even the best of the current energetics or antimatter to enable speeds close enough to that of light for reasonable physical travel over the requisite distances. Therefore, we are up against physics limitations; however, those limitations have uncertainties.
There is a large number of huge unsolved problems in physics, especially at cosmological and interstellar scales. These include the inability to experimentally and directly verify the concepts of dark matter and dark energy, which together constitute some 95% of all matter-energy in the universe. Then there are 120 orders of magnitude discrepancy between the experimental measurements of the cosmological constant and the predictions of quantum theory, which otherwise are usually accurate to many significant figures. Also, we cannot find the massive amounts of antimatter that is supposed to be out there.
We also lack cogent explications for the observed greater than 10,000 times the speed of light effective speed of quantum entanglement, which is the basis of much of the next big little thing beyond nano-quantum technology. In fact, natural quantum entanglement (which is the utilization of materials that are entangled) via the effects of natural or human actions on the other parts of the entangled system alter the operational and safety aspects of space systems.
There are many other issues with physics, especially at cosmological scales. If we understand and can explain these many issues, there may be a possibility that such an altered physical understanding could enable useful human interstellar travel and colonization of the universe.
Major Putative Deep Space Business Cases
Manufacturing
In-space manufacturing has been under study for many years, with considerable associated experimental R&D. The original impetus was the exploitation of microgravity to produce unique products that could be worth the associated additional costs involved compared to terrestrial manufacture. The current litany of such putative unique products from microgravity and vacuum processing include protein, other crystals, improved semiconductors, micro encapsulation, fibers and fiber optics, pharmaceuticals, human organs, and improved metal alloys.
Additionally, in-space manufacturing development has expanded beyond just production in space of terrestrial application products to include reuse and repurposing of space debris, ISRU, real-time mission applications, along with the capabilities proffered by printing fabrication and for both in-space and on-bodies utilization. This task expansion has also included fabrication, assembly, integration, maintenance, repair, and upgrades. Essentially, it’s a combination of in-space and on-body manufacture and application that includes in-service aspects. Advantages of space manufacturing include mission proximity, convection control, no sedimentation, larger and higher quality crystal formation, increased material purity, defect free, and perfect roundness.
Mining
The major item of interest here is water. Like this entire business area, water seriously needs detailed information for mission and business planning. The questions that need to be addressed include what is there, how much is there, and exactly where it is. Take Moon water as a current example: Tests indicate there is indeed water in deep craters at the poles, but there is not yet knowledge of in what form, how much, and exactly where.
Deep space mining can be utilized either for Earth or in space. There are three fundamental approaches for commercial mining in space for use on Earth: Bring the mined material to Earth to be processed, process it in space and then distribute the products, or bring an asteroid to Earth for processing. The economic competition for deep space mining for Earth includes alternative materials utilization, accessing the materials from the seabed or ocean water, and better detection, extraction, and processing of extant availability.
Initial efforts in regard to deep space mining concerned precious metals, notably platinum. There were thoughts that some asteroids might contain serious amounts of this. With its high current value, there was apparently a solid closed business case. Other thoughts related to this included the potential effects on the market price of a large infusion of product, which could alter much the profit outlook. Also, some major uses of platinum are subject to alternative material utilization.
Another approach to determining the possible business case for deep space mining for Earth is to consider the projected materials shortages here. Within the next several decades, antimony shortages are forecast. By the next 100 years, shortages are projected in molybdenum, rhenium, zinc, and gold.
Note the absence here of rare earths, which apparently are not rare, but some ores are easier to access and process than others. Also, there is ongoing material and function substitution with rare earths, which is based upon cost issues. There is an excellent study on the occurrence of rare earths in deep space. They are present in deep space, notably on the Moon and Mars, but are assessed as not being of sufficient concentration to be exploitable. However, higher concentrations might be found near specific craters or locations deposited by an asteroid. That possibility also applies to all deep space materials and is to be determined, depending upon far better definition of what is in deep space, where, and how much of it exists.
Considering the avowed potential scarcities on Earth, molybdenum, zinc, and gold are contained in nodules on the ocean floor and in the ocean water. That leaves antimony, rhenium, and possibly indium. Indium is on the Moon, and Mars has rhenium.
Then there is, as mentioned, water. There has been great interest in mining whatever water is at the poles of the Moon for rocket fuel. NASA computations indicate that it is cheaper to bring water up from Earth than to mine it and bring it from the Moon, especially with reusable rocket econometrics. Space X is projecting launching on starship 100 metric tons for $2 million, hugely cheaper than historic launch costs.
Except for indium and rhenium, deep space mining is apparently primarily for use in deep space for the next century or so.
Deep Space Tourism
Orbital space tourism began with the Russians, who in eight trips and after suitable training, took seven tourists to the International Space Station (ISS) from 2001 to 2009 on Soyuz. The nominal cost of such trips is now in the range of $20 million to $25 million, via Blue Origin, Virgin Galactic, Boeing, and Space X. The U.S. is planning for Space X Crew Dragon and Boeing Starliner to transport tourists (sometimes termed spacecraft participants) to the ISS.
NASA is largely responsible for the reinvigorated emphasis upon private human space travel. The NASA commercial crew program with Boeing and Space X has as one of its objectives fostering commercially supplied private human space access. With that program thus far a success, there are now serious plans to exploit that commercial space access capability for space tourism. Bigelow Aerospace and Orion Span have nascent luxury orbital hotel designs. Space X and Space Adventures plan Moon loop flights. A survey indicates that there is significant public interest in spending up to two weeks on the ISS with a spacewalk, and some are desirous of a space hotel.
Overall, there appears to be accelerating serious near‐term interest in orbital space tourism. The current costs are, for most, daunting, but the activity, interest, and now emerging capabilities to do these trips, plus the large Space X low-Earth-orbit (LEO) access cost reductions, bode well for the future of space tourism (providing that there are no accidents and loss of life). The projected mean number of missions for loss of crew on the space shuttle was a factor of order two more than what was observed. However, the shuttle was designed in the 1970s and built for half the projected cost due to budget cuts. We know more now. Therefore, space tourism will probably experience at least the historical average on the order of 100 flights between failures. The extent to which rocket safety can be improved and its public acceptance is to be determined.
Serious space tourism is starting in Earth orbit over the next few years. Two weeks or less in space, from the available information, should not produce many of the adverse health effects that characterize longer stays. ISS is within the planetary magnetic fields that offer some protection from radiation (even though it’s above the protective atmosphere). In fact, the radiation exposure is half of that which would be received in deep space (e.g., during trips to the Moon, Mars, and other destinations). Typical ISS duty periods for highly trained ISS astronauts are six months. In deep space with exposure to full radiation, serious radiation protection and artificial gravity will be needed for Martian trips and beyond.
With respect to the present status of virtual or digital space tourism, NASA has a number of immersive virtual tours of destinations such as the ISS and the planets. Companies offer virtual tours of the Moon, Mars, the planets, and the solar system. Google offers a tour of the ISS, space tours are on YouTube, and virtual reality companies are planning to offer live virtual tours of Earth from space. Digital reality will increasingly provide space tourism experiences that are some million times less expense and some thousand times safer.
After 60 years of highly trained astronauts going into space, space related technologies have advanced. Their costs have reduced to the point where increasing numbers of private citizens can become space tourists. The safety aspects of physical space tourism, including the human health concerns, need further development. However, those that relate to the space environment are tolerable for a few weeks based on the 60 years of manned space flight experience. As space tourism expands beyond Earth orbit to the Moon, Mars, asteroids, and other planets, the safety issues will need to be seriously worked, and that is doable.
ISRU/Space Colonization
Space colonization is first order dependent on body resources, efficient ISRU, and energy, even if colonization sites are in space. The costs of serious resupply from Earth for sizable space colonies are probably prohibitive. The initial body of interest for colonization is Mars, due to its exceedingly rich resources. The Moon is perhaps next in line due to its proximity to Earth and the presence of some resources, although the amount of the most important Moon resource (water) is currently unknown.
ISRU can provide habitats, return fuel, on-planet fuel, entry-descent landing fuel, life support (e.g., water, O2, atmospheric N2, food, thermalization, pressurization, dust protection), radiation protection, equipage via printing from regolith minerals, volatile extraction, synthetic biology/bio fabrication, and printed plastics derived from H2O and CO2. Some notable advantages of ISRU include fuel production for ascent and consumables, reduced initial mass in low Earth orbit and reduced number of launches, reduced lander size and volume, greater surface exploration capability, life support redundancy, lower risk via fewer launches, and lower life cycle cost.
The currently known resources on Mars are massive, including extensive quantities of water and CO2 and therefore C, H2, and O2 for life support, fuels, plastics, and much else. Mars has very little water in the atmosphere, but estimates indicate much of the water that was ever on Mars is still there. Massive amounts of water ice in the north pole region, many kilometers thick, is enough to put an ocean on a flat Mars many meters deep.
There are major quantities of water ice and water adsorbed on minerals in the regolith. From 3% at the equator (up to 8% localized) to 40% plus at 60 degrees north, we can microwave heat it to produce water vapor, capture, and store it as ice in container. A solar tent could produce sufficient heat to vaporize the regolith water ice.
Water is also available from sulfates (22% H2O), silicates, etc. A large crater near the equator purportedly has an ice lake the size of Lake Huron, with similar or greater depth, and there have been discoveries of large ice lakes near the pole. CO2 is available from deposition on exposed cooled surfaces. The regolith is replete with all manner of minerals.
ISRU applicable frontier technologies include robotics, machine intelligence, nanotechnology, synthetic biology, 3D printing and additive manufacturing, and autonomy. These technologies, combined with the vast natural resources, should enable serious pre- and post-human arrival ISRU to greatly increase reliability and safety and reduce cost for human colonization of Mars.
Various system-level transportation concepts employing Mars-produced fuel would enable Mars resources to evolve into a primary center of trade for the solar system for eventually nearly everything required for space faring and colonization. Mars resources and their exploitation via extensive ISRU are the key to a viable, long-term, safe, and affordable human presence beyond Earth.
The major currently envisaged destinations for human missions and colonization include the Moon, Mars, poles of Mercury, upper atmosphere of Venus, Europa, Callisto, Enceladus, Titan, and Triton. The evaluative metrics for these destinations include temperature, radiation, water, atmospheric pressure, minerals, organics, volatiles, solar energy, atmospheric composition, and lava tubes, among many others.
All these destinations have water except Venus, where hydrogen could be extracted from the sulfuric acid rain and combined with oxygen from the CO2 atmosphere. Venus uniquely has Earth-like gravity, temperature, pressure, and low radiation when living 50 km above the surface and floating in the atmosphere.
After Mars and Venus, the next most salubrious destination for humans is Titan with its low temperature and gravity, but with low radiation, high pressure, and organic lakes. Callisto also has low radiation, but shares cold temperatures, low gravity, and near vacuum pressure with the other destinations. All have minerals and at least some organics. Those destinations beyond Mars have low solar energy. Titan has copious hydrocarbons. The asteroids purportedly have precious metals and water. The required radiation protection on bodies is readily available via living, working under some four to five meters of regolith with such living quarters produced via ditch and bury or utilization of lava tubes as caverns.
Traveling beyond Mars would require use of nuclear power via batteries or fission reactors, with the goal of using fusion propulsion. Ongoing reductions in costs for space access will enable development of serious commercial deep space beyond GEO, eventually at the trillion dollar a year level. The technologies and infrastructures developed for commercial deep space will be efficacious for human solar system missions and colonization.
Services
There are a plethora of services for deep space development, which constitute an additional major space business arena. Communications is a current example. Others include on-orbit servicing, assembly, and manufacturing and space general contracting services, fuel depots and fuel supply, life support supplies (water, food, O2, etc.), repair services, and transportation of many flavors.
Over the next few decades, the development and buildout of commercial deep space and space colonization will require:
- Solutions to space debris
- Solutions to and mitigation of the many space‐related human health issues, the foremost of which is radiation protection
- Inexpensive space access, in progress
- Reliability and safety improvements
- In-space and on-body resources mapped in detail
- Advanced power and energy options researched, developed, demonstrated, including fission, advanced radiologic batteries, and positrons
- Closed commercial deep space business plans, possible due to inexpensive space access
- Legalities solved regarding both space debris and on-body resources, becoming ever more critical as deep space mining becomes real and the debris problem worsens
- Trusted AI developed, applied to reduce costs of deep space development via autonomous robotics
- Moon and Mars dust control, a potential serious health and operability issue
- Optimization of ISRU writ large, including via AI and robotics
Martian colonization has moved from extremely difficult to increasingly feasible in regard to radiation, cost, and safety. There are now several ways forward to affordable and safe humans-Mars missions because of reusable rockets, serious ISRU, energetics/fast transits, achievable radiation protection, AI, robotics, printing, and ever greater knowledge of Martian resources, along with a solution for space debris.
About the Author
Dennis M. Bushnell is chief scientist at NASA Langley Research Center in Hampton, Virginia. This article draws from and updates “Futures of Deep Space Exploration, Commercialization, and Colonization: The Frontiers of the Responsibly Imaginable” (March 2021), the author’s technical memorandum for NASA’s scientific and technical information program.
Image above: NASA's Mars missions, clockwise from top left: Perseverance rover and Ingenuity Mars Helicopter, InSight lander, Odyssey orbiter, MAVEN orbiter, Curiosity rover, and Mars Reconnaissance Orbiter. Credit: NASA/JPL-Caltech